LIGO Detects Merging Ripples in Space and Time
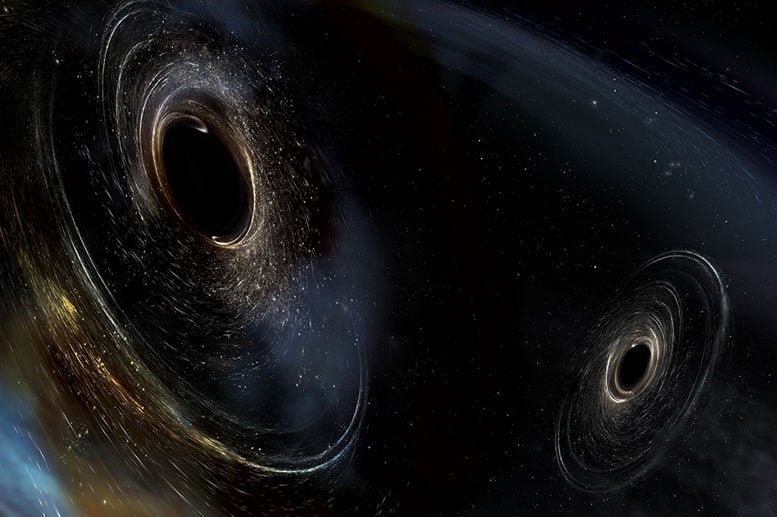
This
artist’s conception shows two merging black holes similar to those
detected by LIGO. The black holes are spinning in a nonaligned fashion,
which means they have different orientations relative to the overall
orbital motion of the pair. LIGO found hints that at least one black
hole in the system called GW170104 was nonaligned with its orbital
motion before it merged with its partner. Image: LIGO/Caltech/MIT/Sonoma
State (Aurore Simonnet)
The collision of a pair of colossal, stellar-mass black holes has made itself heard, nearly 3 billion light years away, through a cosmic microphone on Earth.
On January 4, the Laser Interferometry Gravitational-wave Observatory (LIGO) picked up a barely perceptible signal that scientists quickly determined to be a gravitational wave — a ripple of energy passing through the curvature of spacetime. The event, published today in Physical Review Letters, marks the third direct detection of a gravitational wave.
Catalogued as GW170104, the signal, when translated into the audio band, resembles an upward-sweeping chirp, characteristic of a “binary coalescence,” or a merging of two massive astrophysical objects in the distant universe. The team has concluded that the gravitational wave was produced by the collision of two heavy, stellar–mass black holes, one estimated to be about 31 times, and the other 19 times, as massive as the sun.
The signal captured by LIGO lasts less than two-tenths of a second, and in that fraction of a moment, scientists calculate that the black holes whirled around each other about six times before merging into one giant, 49-solar-mass black hole. This cosmic collision gave off an enormous amount of energy in the form of gravitational waves, equivalent to two times the mass of the sun.
The merger took place about 3 billion light years from Earth, measuring about twice as far as the black hole collision that produced GW150914, LIGO’s first-ever gravitational wave detection.
“This is indeed the farthest out stellar-mass black hole system anyone has seen,” says Erik Katsavounidis, senior research scientist in MIT’s Kavli Institute for Astrophysics and Space Research and a member of the LIGO team.
A mathematical calculation of the warped spacetime near two merging black holes. The simulation is consistent with LIGO’s observation of the event dubbed GW170104. The colored bands are gravitational-wave peaks and troughs, with the colors getting brighter as the wave amplitude increases. The throats near the center of the movie indicate the strong spacetime warping near the black holes’ event horizons. Credit: SXS Collaboration.
Out of alignment
The new gravitational wave signal is similar to LIGO’s first two detections, both in its source — a binary black hole merger — and the overall mass of that source.
However, the scientists discovered an interesting feature in the newest signal: The spin of at least one of the black holes may have been “antialigned” with the orbital angular momentum — the direction in which the black holes were orbiting each other. This phenomenon would be similar to teacups spinning counterclockwise on a clockwise-rotating carnival platform.
Katsavounidis stresses that the signs for antialignment are small, though potentially significant. If scientists detect more antialigned systems, such evidence may support a formation scenario known as dynamical capture, in which black holes evolve separately in a cosmic environment cluttered with stellar objects. In such an environment, black holes with various spins can eventually pair up in binary systems, simply through gravitational, “dynamic” attraction.
Dynamical capture runs counter to a model called “common envelope evolution,” in which binary black holes evolve together, with spins that are aligned with their orbital angular momentum. In fact, the LIGO team inferred that the December 2015 detection had a strong probability of aligned spins, contrary to this newest signal.
“Here for the first time, we’re seeing antialignment is favored,” Katsavounidis says. “If we can detect more systems, we can nail down under what circumstances black holes formed and evolved to form binary systems that ultimately merged.”
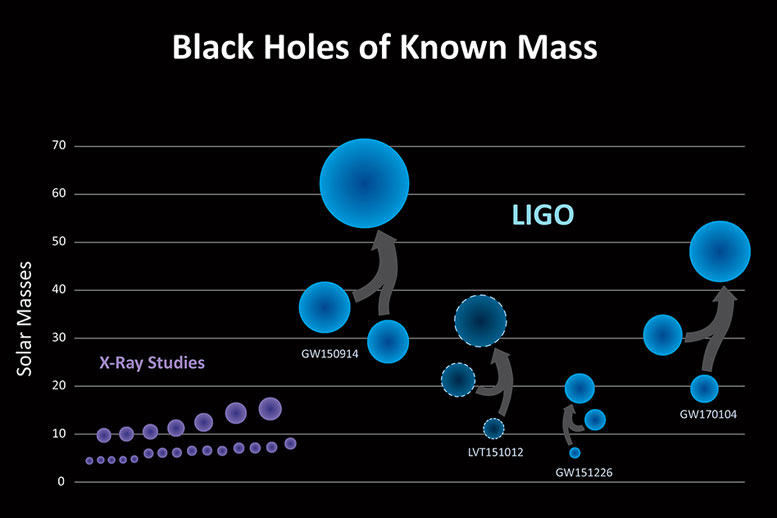
LIGO
has discovered a new population of black holes with masses that are
larger than what had been seen before with X-ray studies alone (purple).
The three confirmed detections by LIGO (GW150914, GW151226, GW170104),
and one lower-confidence detection (LVT151012), point to a population of
stellar-mass binary black holes that, once merged, are larger than 20
solar masses. Image: LIGO/Caltech/MIT/Sonoma State (Aurore Simonnet)
After undergoing tune-ups to improve its sensitivity, LIGO began its second observing run on November 30, 2016. Katsavounidis says GW170104’s detection had “a certain aspect of serendipity.”
On January 4, 2017, at 10:11:58.6 UTC, a gravitational ripple was recorded passing through one of LIGO’s detectors, in Hanford, Washington. Three milliseconds later it passed through the twin detector more than 3,000 kilometers away in Livingston, Louisiana. The ripple caused each detector to alternately expand and shrink ever so slightly, generating a small wiggle in the data gathered by both detectors.
Within tens of seconds, LIGO’s search algorithms automatically analyzed the signal, comparing it to waveforms characteristic of gravitational waves.
“A very careful researcher in Germany was looking at the data as they were coming in, and noticed one of the two detectors picked up something significant,” Katsavounidis says. “That event was identified in near-real time, thanks to that colleague.”
The researcher immediately notified LIGO’s detector operations, characterization, and data analysis working groups, which set to work further dissecting the signal. The scientists used computational tools to narrow in on a likely set of parameters, such as a system’s mass, spin, and orientation, that would produce a gravitational signal matching the one seen in the data.
The best fit turned out to be a pair of merging black holes, which the scientists calculated to be the second most massive stellar-mass binary black hole system, behind GW150914, LIGO’s first gravitational wave detection.
Fighting gravitational fuzziness
With this new detection, the team again confirmed Albert Einstein’s theory of general relativity, observing that the behavior of the merging black holes agreed with Einstein’s predictions of gravitational effects, even at such extreme scales.
“That’s an amazing thing,” Katsavounidis says. “Whether you talk about gravity on Earth, or something where the gravitational potential is a billion times greater, general relativity still describes how those gravitational waves are generated and how those objects behave gravitationally.”
As part of the initial analysis of the signal, LIGO researchers produced “sky maps” with approximate areas in the sky for where the binary black hole system might be located. As part of its standard procedure, LIGO sent these sky maps out to about 80 partner astronomy groups, each of which has access to imaging tools that span the entire electromagnetic spectrum, as well as neutrinos. While LIGO continues to listen for signs of other extreme events in the universe, astronomers have been pointing their telescopes in the direction of GW170104’s source, hoping to see glimmers of the colliding black holes.
“LIGO acts as our ears, so to speak, and we want to listen for something and quickly move our eyes to follow the signal,” Katsavounidis says. “Our mission is to fight the fuzziness of gravitational wave detectors by adding more of them in the global network, and by pairing [the detections] with light as soon as possible.”
The search for gravitational waves will soon gain an additional set of ears, in the form of Virgo, a similar detector located near Pisa, Italy, that is scheduled to come online this summer and will pair with LIGO.
“Coming from a field of looking for something rare, I’ve always been hesitant, with one detection only, to declare victory,” Katsavounidis says. “I can tell you I’ve started sleeping much better after the second detection. Now this third one solidifies LIGO and LIGO’s observations as the ultimate tool to see the mass spectrum of black holes in our universe.”
“With the third confirmed detection of gravitational waves from the collision of two black holes, LIGO is establishing itself as a powerful observatory for revealing the dark side of the universe,” says David Reitze of Caltech, executive director of the LIGO Laboratory. “While LIGO is uniquely suited to observing these types of events, we hope to see other types of astrophysical events soon, such as the violent collision of two neutron stars.”
This research was supported, in part, by the National Science Foundation.
Publication: B. P. Abbott et al. (LIGO Scientific and Virgo Collaboration), “GW170104: Observation of a 50-Solar-Mass Binary Black Hole Coalescence at Redshift 0.2,” Phys. Rev. Lett. 118, 221101, 2017; doi:10.1103/PhysRevLett.118.221101
Source: Jennifer Chu, MIT News
Your Opinion is valid .